Low-Energy Extraction Wells a Cheaper Option? : IN DEPTH: Groundwater Monitoring of Landfill Leachate
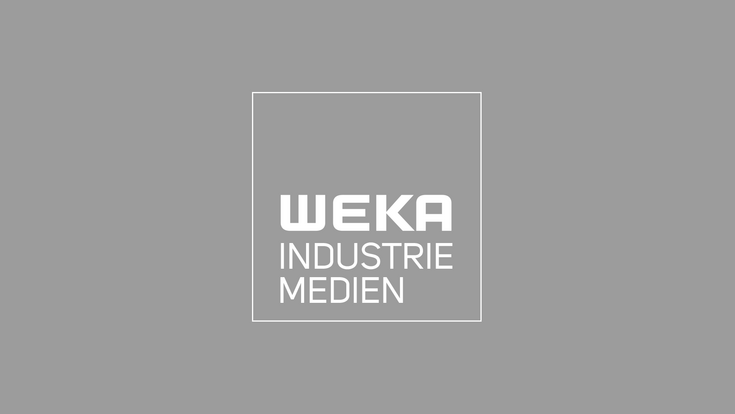
Early detection of contaminants in groundwater released from landfill sites helps facilitate timely and effective source control and mitigation, but could low-energy extraction wells offer a cheaper alternative to passive wells?
Over the past three decades, extensive research has been conducted on detecting and remediating groundwater pollution from various sources. Landfills and other waste storage facilities often contaminate soil and groundwater. Over long time periods, monitoring and mitigating groundwater contamination are potentially costly endeavours.
Effective detection monitoring programs take into account the unknown locations of possible contaminant releases, directions of groundwater flow, the characteristics of subsurface material, and locations of neighbouring properties and potential receptors of polluted groundwater.
Besides using groundwater wells, which only detect contaminants after a release from a waste storage facility, a pan lysimeter or other vadose or unsaturated zone monitoring system may confirm liner leakage before widespread groundwater pollution occurs. However, samples from wells in the saturated zone are necessary to confirm groundwater contamination and help inform remedial action.
Often, the objective of groundwater monitoring systems at waste storage sites is to detect contaminant plumes before they reach a regulatory compliance boundary to allow timely mitigation. Generally, wider contaminant plumes, influenced by such factors as groundwater seepage velocity and transverse dispersivity, tend to be easier to detect than narrower plumes.
Conventional detection wells are passive receptors; they detect contamination if a plume passing through them is sampled and analysed. Chloride sensors or polymer-based microsensors for volatile organic compounds enable continuous online monitoring and may enable timely detection if placed in strategically located, passive wells. Such techniques complement periodic sampling and analysis of specific contaminants to detect and characterize plumes.
Fitting detection wells with pumps extracting water at very low rates – sustainable with inexpensive and renewable energy sources – may be a viable alternative to traditional monitoring. Actively drawing in water, fewer low-energy wells could potentially detect contaminants emerging from the same source area as several passive wells. Depending on their location, low-energy wells may also help remediate contaminated groundwater.
While capable of containing and removing contaminant plumes in some settings, previous literature does not address the use of low-energy wells for detecting releases, especially narrow plumes emerging from small source areas that are difficult to detect. Yet, point sources such as holes, tears, or imperfections in a liner are more common than broad source areas at contemporary landfills.
Small source areas produce contaminant plumes moving as fingers, with limited lateral spread between the landfill and point of compliance. The objective of this study was to model and compare low-energy extraction wells with conventional passive networks for detecting contaminants released from small source areas in landfills. Simulations involved low discharge rates, attainable with solar power in some regions.
METHODS
Modular Three-Dimensional Multispecies Transport Models (MT3DMS) were used to simulate groundwater flow and mass transport in a hypothetical unconfined aquifer (Figure 1). The model involved a block-centred, finite-difference grid of 830 columns, 300 rows, and one layer with a node spacing of 0.1 m along rows (oriented east-west) and columns (oriented north-south).
Water table elevation was 5 m at the western boundary and 4.17 m at the eastern boundary of the model, producing an average hydraulic gradient of 0.01 eastward. The aquifer’s lower boundary had a 0 m elevation. No groundwater crossed the northern, southern, or lower boundaries of the model.
Other model parameters were a hydraulic conductivity of 1.0 m/d, effective porosity of 0.25, longitudinal dispersivity of 1.0 m, transverse dispersivity of 0.1 m, and effective molecular diffusion coefficient of 0.00001 m2/d.
The model simulated contaminants released from all 151 nodes along a 15 m source segment oriented perpendicular to eastward groundwater flow (Figure 1). Each point source had an area of 0.01 m2 and constant concentration of 100 mg/L. Through numerous simulations, the model identified the minimum pumping rate required for one extraction well, located 5 m downgradient of the midpoint of the source segment, to detect releases from all nodes.
A conservatively small offset of 5 m was chosen, because contaminant plumes in groundwater tend to undergo less widening with hydrodynamic dispersion over shorter distances of travel.
Next, the model identified the minimum number and locations of passive wells necessary to detect all releases along the 15 m transect. Passive wells also occupied a monitoring transect, perpendicular to groundwater flow, located 5 m downgradient of the source segment.
The boundary of each contaminant plume had a concentration of 1 mg/L. Detected plumes passed through the low-energy well or a passive well prior to reaching the eastern model boundary; that is, the concentration at the low-energy well or a passive well equalled or exceeded 1 mg/L before the concentration at any node along the eastern boundary reached 1 mg/L.
Both passive and low-energy wells were open to the saturated thickness of the aquifer in model simulations. All groundwater flow and mass transport simulations used the preconditioned conjugate gradient and generalized conjugate gradient solvers, respectively, yielding mass balance errors of less than 1%.
RESULTS
One low-energy well extracting groundwater at 1.1 m3/d detected releases from all point sources along the source segment. The effect of the well can be seen by examining the geometry of a contaminant plume originating at the northern end of the source segment.
After 1600 days, the contaminant plume reaches the downgradient boundary, but not until the concentration reaches 1.0 mg/L at the extraction well. The plume bends slightly southward, enabling a well not directly in its path to still register a detection.
In contrast, a minimum of three passive wells identified all releases from the same point sources. The passive wells have negligible effect on the groundwater flow field; more passive wells were necessary, because they have less capability to draw in contaminated groundwater.
After 1300 days, a contaminant plume originating at the source reaches the downgradient boundary, but after the concentration at one or more passive wells reaches 1 mg/L. This result shows that passive wells may be useful when strategically placed and monitored, even for narrow contaminant plumes that have travelled short distances. However, a tendency for hydrodynamic dispersion to widen contaminant plumes with travel enhances the capability of both passive and low-energy extraction wells to detect them.
Overall, fewer low-energy wells than passive wells were necessary to detect all source nodes. While this example involved a relatively small study area, resulting in a 3:1 ratio of passive wells to low-energy wells, larger sites with a similar ratio would increase the absolute difference between well types, resulting in more cost savings in drilling and sampling when using low-energy wells.
Low-energy wells decrease the possibility of excessive drawdown and dewatering sometimes encountered with higher capacity wells and, if strategically placed, may perform a dual role in remediating contamination.
Results outlined above suggest that low-energy extraction wells may be a viable approach for detection monitoring at some waste storage facilities. However, they would not be equally effective in all settings. For example, low-energy extraction wells might produce very narrow capture zones in fast-moving groundwater. Such conditions might also produce narrow contaminant plumes, which are more difficult to detect with few low-energy or passive wells, but using more wells could address this problem.
CONCLUSION
The objective was to evaluate low-energy extraction wells as an alternative to passive well networks for detecting contaminants from landfills. Results suggest that, even with very low pumping rates, extraction wells can effectively reduce the number of sampling points required to detect contamination in groundwater. In some cases, low-energy wells may be a viable, less costly alternative to conventional monitoring wells.