New research on landfill leachates : How detection wells converted to low-discharge pumping wells can help stabilise leachate plumes from landfills
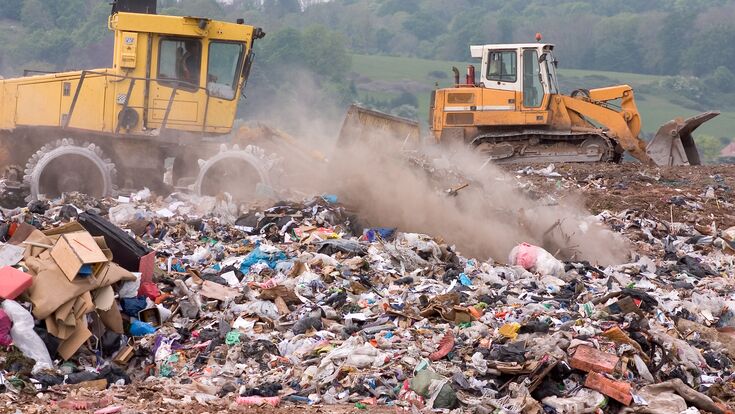
Abstract
An approach for converting passive detection wells to low-discharge pumping wells, to stabilize narrow leachate plumes, was developed and evaluated. A mass transport model simulated plumes from point sources in a hypothetical landfill above an unconfined alluvial aquifer. All plumes passed through at least one well in an initial detection network but reached the site boundary without active remediation. In subsequent simulations, after a plume was first detected, the detecting well was converted to extraction, and the farthest cross-gradient wells were converted to injection, each pumping at half the rate of the extraction well. Minimum extraction rates required to stabilize plumes onsite ranged from 0.3 m3/d to 0.5 m3/d. Converting passive detection wells to low-discharge pumping wells may stabilize narrow plumes in some cases, giving landfill managers time to evaluate and implement remedial options.
Introduction
Unlined landfills often produce leachate that contaminates soil and groundwater. Leachate can also seep through small holes or tears in lined landfills. At breached landfills, timely and effective source control and aquifer remediation reduce pollution threats to nearby supply wells and other groundwater receptors. Preventing leachate plumes from moving offsite is a top priority for landfill managers. Measures that slow or stop plume growth give landfill managers time to evaluate natural attenuation and other remedial options. Emerging sustainable technologies for remediating soil and groundwater contaminated by landfills include permeable reactive barriers, electrokinetic methods, microbial action, and injecting solubilizing agents or air bubbles (Ye et al., 2019).
Upon documenting a breach, low-discharge wells powered with solar energy may be useful for slowing or stopping plume growth. Groundwater removed can be treated above ground and injected back into the subsurface. Small holes in landfill liners tend to produce narrow contaminant plumes that are potentially recoverable with low-discharge wells. Moreover, it may be possible to convert passive detection wells, already required at landfills, to low-discharge pumping wells to stabilize a contaminant plume. This article outlines and evaluates a strategy for converting detection wells to pumping wells, to stabilize narrow contaminant plumes from a hypothetical lined landfill.
Related article: New Waste Management Standard Will Aid in Soil and Biosolid Analysis
Methods
A graphical approach (Hudak, 1998) was used to design a passive detection monitoring network for a hypothetical landfill (Figure 1). Flow tubes of equal width were overlaid on the footprint of the landfill. Monitoring wells occupied centerlines of flow tubes, 10 m downgradient of the landfill. A mass transport model (Zheng and Wang, 1999) was used to find the maximum width of flow tubes (minimum number of wells) required to detect a contaminant plume originating from any point source within the landfill.
The finite-difference model comprised one layer, 570 columns (trending north-south), 340 rows (trending east-west), and 193,800 cells. Nodes at cell centres were 0.50 m apart along columns and rows. The hydraulic head was 5.000 m and 2.155 m at the farthest west and east columns, respectively, measured from a datum at the base of the model. No flow moved across the northern or southern boundaries. Eastward, the hydraulic gradient averaged 0.01.
Other variables, representative of an alluvial aquifer (API, 1989), were a hydraulic conductivity of 1.0 m/day, effective porosity of 0.25, longitudinal dispersivity of 1.0 m, transverse dispersivity of 0.1 m, and effective molecular diffusion coefficient of 0.00001 m2/day. In mass transport simulations, point sources had a concentration of 100 mg/L. Plume boundaries had a concentration of 1.0 mg/L. Simulations used the preconditioned conjugate gradient solver for flow and generalized conjugate gradient solver for mass transport. Mass balance errors for flow and transport simulations were less than 0.03%.
After establishing an initial detection network, the model generated contaminant plumes emerging from five random point sources (one at a time) within the footprint of the landfill. Model output was studied to identify plume geometry at the time first detected, and the time first contacting the site boundary.
The source remained on in subsequent remediation trials. When a contaminant plume first reached a detection well, that well was converted to an extraction well, and the farthest cross-gradient wells were converted to injection wells. Each injection well pumped at half the rate of the extraction well, but in the opposite direction. Through multiple simulations with different pumping rates, the model output identified the lowest pumping rate required to stabilize a plume onsite. Stability meant the leading tip of the plume stopped advancing, as the source and pumping wells remained active. Model output also identified plume geometry at stability and the amount of time to achieve stability.
Results and Discussion
The initial detection network consisted of 12 monitoring wells near the two downgradient sides of the landfill (Figure 1). Five random sources occupied various points inside the landfill’s footprint (Figure 2). In separate simulations, from each point source, a narrow contaminant plume developed and reached one or more detection wells before reaching the downgradient site boundary. Contaminant plumes were first detected between 270 days (Case 4) and 720 days (Case 5) (Table 1, Figure 2). Plumes reached the site boundary between 4,000 days (Case 4) and 4,800 days (Case 3) (Table 1, Figure 2).
The contaminant plume in Case 4, closest to the landfill’s downgradient boundary, was detected relatively early, resulting in the timely conversion of detection wells to pumping wells. Additionally, the plume in Case 4 was relatively small at detection; therefore, it took relatively less time to stabilize with pumping (Table 1).
Case | Time to first well (d) | Time to site boundary (d) | Minimum pumping rate (m3/d) | Time to stabilize (d) |
---|---|---|---|---|
1 | 680 | 4560 | 0.4 | 5400 |
2 | 510 | 4160 | 0.4 | 4200 |
3 | 690 | 4800 | 0.4 | 3200 |
4 | 270 | 4000 | 0.3 | 3500 |
5 | 720 | 4780 | 0.5 | 3900 |
In contrast, sources in Cases 1 and 5 were further upgradient in the landfill and thus larger when associated plumes were first detected. The plume in Case 5 was slightly larger and required a higher pumping rate, which stabilized the plume in less time (compared to Case 1). Shortening time to stabilization, the converted extraction well in Case 5 was better aligned, along the ambient hydraulic gradient, with its associated leak, thereby targeting higher contaminant concentrations (Table 1, Figure 2).
The point source in Case 3 was also closely aligned, along the ambient hydraulic gradient, with the first well (a cross-gradient well) detecting the plume. Because one cross-gradient well was converted to extraction, only one cross-gradient well remained, which was converted to injection – at the same rate as the extraction well, but in the opposite direction. This variation worked effectively, as the associated plume stabilized relatively early and was relatively small at stabilization.
The minimum pumping rate required to stabilize a plume ranged from 0.3 m3/d (Case 4) to 0.5 m3/d (Case 5) (Table 1, Figure 2). Case 4 took less time to stabilize because the plume was small at first detection, and the converted extraction well nearly aligned (along the ambient hydraulic gradient) with the source. Early detection and effective alignment in Case 4 also resulted in a small plume at stabilization (Figure 2).
After pumping began, the time to stabilize a plume ranged from 3,200 days (Case 3) to 5,400 days (Case 1) (Table 1). Plumes grew slowly toward stable geometry but did not cross the site boundary. At stability, plumes were the narrowest downgradient of the extraction well, which pulled in contaminants that otherwise would widen the plume (Figure 2). Meanwhile, cross-gradient injection wells pushed contaminants toward the extraction well, thereby contributing to plume narrowing. The stable, leading tip of a stabilized plume reflects a balance between the competing effects of the extraction well and ambient hydraulic gradient. At stabilization, the highest concentrations are found in a localized area around the source and downgradient segment of the landfill boundary (Figure 2).
In practice, stabilizing a contaminant plume prevents offsite migration while giving landfill managers time to assess a situation and apply appropriate remediation measures. Remedial action might involve repairing a leak, monitoring the natural attenuation of a leak and associated plume, or additional measures outside the footprint of a landfill to reduce the size of a plume. While potentially effective in some cases, low-discharge pumping wells would not work as effectively for contaminants with low solubility, or for wide contaminant plumes.
The cases outlined above involved modelling a contaminant plume at first detection, to find a minimum pumping rate to stabilize it on site. However, in practice, the location of a point source, and the geometry of an associated plume, are rarely known at first detection. Samples from an initial array of detection wells can aid in defining a plume. The location of the first well that detects a contaminant plume helps narrow down a plausible (upgradient) subarea containing the point source. Additional wells and samples may be necessary to define an initial plume for modelling remedial action.
Conclusion
This study examined the capability of passive detection wells converted to low-discharge wells for stabilizing narrow leachate plumes originating from lined waste impoundments. The first well contacting a plume was converted to extraction, and the farthest cross-gradient wells on either side of the extraction well were converted to injection. At low pumping rates, this conversion strategy effectively stabilized five plumes emerging from small random leaks in a hypothetical landfill. The strategy outlined here may be worthwhile at some sites contaminated by small leaks in lined impoundments.
References
API (American Petroleum Institute) (1989). Hydrogeologic Database for Groundwater Modeling. American Petroleum Institute, Washington, D.C.
Hudak, P.F. (1998). Configuring detection wells near landfills. Ground Water Monitoring and Remediation, 18(2), 9396.
Ye, J., Chen, X., Chen, C., Bate, B. (2019). Emerging sustainable technologies for remediation of soils and groundwater in a municipal solid waste landfill site – A review. Chemosphere, 227, 681-702,
Zheng, C., Wang, P.P. (1999). MT3DMS: A Modular Three-Dimensional Multi-Species Transport Model for Simulation of Advection, Dispersion, and Chemical Reactions of Contaminants in Groundwater Systems; Documentation and User’s Guide. U.S. Army Engineer Research and Development Center, U.S. Army Corps of Engineers, Contract Report SERDP-99-1, Vicksburg, Mississippi.