CCUS : Carbon Capture: How to catch a gas
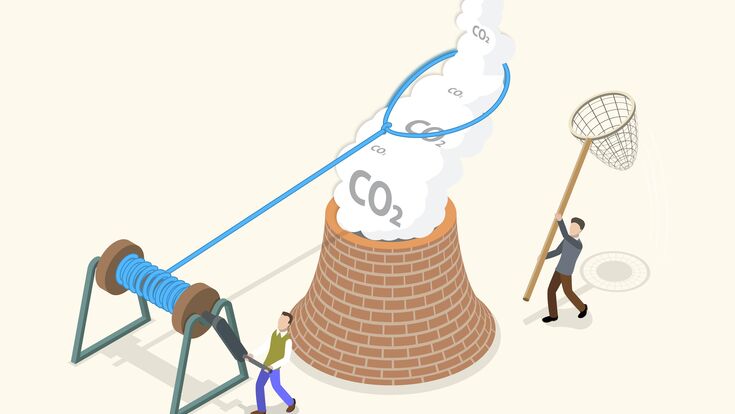
How can we reduce CO2 emissions? That is, of course, the key question in the ongoing discussion on the mitigation of climate change. To achieve net zero, the development and exploitation of decarbonisation technologies need to be pushed. Quite a few experts see carbon capture and storage (CCS) as well as carbon capture and usage (CCU) as essential in this effort.
In its Sustainable Development Scenario, the International Energy Agency (IEA) defines a pathway to the outcomes targeted by the Paris Agreement, in which global CO2
emissions from the energy sector fall to zero on a net basis by 2070. In total, 15% of the global emissions reduction is delivered by carbon capture, utilisation and storage (CCUS). “The contribution of CCUS grows over time and extends to almost all parts of the global energy system,” the IEA stated. But to be able to achieve this, the installed capacity of CCUS needs to increase one hundredfold by 2050. “While challenging, this target is within reach with supportive policy development that addresses regulatory concerns and shores up a supportive environment for CCS investment,” says Bruno Gerrits of the Global CCS Institute, an international think tank whose mission is to accelerate the deployment of CCS.
In 2021, 27 CCUS projects were in operation, 5 under construction, 66 in advanced development and 97 announced or in the early stages. (see Figure 1)
A godsend for the heavy industry?
CCUS involves the capture of CO2 from large point sources, including power generation or industrial facilities that use either biomass or fossil fuels for fuel. “With its versatile application, we’re seeing carbon capture and storage being applied to a broad number of sectors,” says Gerrits. “While CCS has been historically associated with the energy sector, the technology is now being used to reduce CO2 in heavy industry across the spectrum, including iron and steel, cement and chemical production.” According to Gerrits, the natural gas industry, along with ethanol production and power generation, is seeing notable CCS growth and investments. In addition to the commercial CCUS facilities operating around the world, there are also a large number of CCUS pilot or demonstration projects as well as projects in earlier stages of development.
According to the Sustainable Development Scenario, CCUS accounts for between one quarter and two thirds of the cumulative emissions reductions in heavy industry (steel, cement and chemicals production): “By 2070, nearly half of global energy demand for aviation is met by synthetic fuels, requiring the capture of around 830 Mt of CO2 for use as feedstock.”
The Sustainable Development Scenario also shows that an initial focus of CCUS is on retrofitting fossil fuel-based power and industrial plants. “By 2030, more than half of the CO2 captured is from retrofitted existing assets,” the IEA states. “CCUS can be retrofitted to existing power and industrial plants that could otherwise emit 600 billion tonnes of CO2 over the next five decades – almost 17 years’ worth of current annual emissions.”
Various technologies
CCUS refers to a variety of technologies, such as chemical absorption, physical, oxy-fuel, membrane or direct separation, calcium or chemical looping and supercritical CO2
power cycles, with chemical absorption and physical separation being the most advanced and widely adopted capture technologies.
These technologies are at different stages of development. “For example, amine solvents circulating in large columns to capture and consequently release purified CO2 are already applied in a waste-to-energy (WtE) context, such as at the AVR WtE plant at Duiven in the Netherlands,” says Tom Croymans, Chairman of the ESWET CCUS working group. “In terms of technology development, a lot of R&D is ongoing and new initiatives are popping up every day. New solvents are being developed aiming to reduce the energy penalty and OPEX of the CC process. Alternatives to the conventional column set-up are being explored on pilot levels, aiming to reduce the footprint and CAPEX of these installations.”
If you want to read the whole interview with ESWETs Tom Croymans, click here!
CCUS projects for waste-to-energy plants are taking off in Europe. “With regard to CCU, the Duiven plant in the Netherlands is already in operation, capturing CO2 and providing it to local greenhouses,” Croymans explains. “For CCS, at least four projects are fairly advanced: the Klemetsrud plant (Norway, part of the Longship project), the Twence plant (Netherlands), which should start operations in 2023, the Amager Resource Centre (Denmark), which should be fully operational by 2025, and the Rakkestad plant (Norway) which is still in the trial phase of the project. Other pilots and projects are also in development, for example in France and the UK.”
While CCS has been historically associated with the energy sector, the technology is now being used to reduce CO2 in heavy industry across the spectrum, including iron and steel, cement and chemical production.Bruno Gerrits, CCS Institute
Commercially feasible
is the more expensive option because of the costs associated with the required further refinement of the CO2,” explains Jens Jørsboe, a researcher from DTU. “So the owners of the Amager Bakke plant are planning to apply for 1.5 billion DKK (230 million USD) for a CCS plant capable of capturing 500,000 tonnes of CO2 per year – if the right regulatory framework and sufficient funding is provided by the Danish state. This plant would employ the same amine scrubbing process that has been proven by the pilot carbon capture plant.”
Looking forward, Jørsboe believes that this technology could be applied at every waste incinerator in the world, representing around 2,500 WtE plants with a disposal capacity of around 400 million tonnes of waste per year.
Interesting topic? For further opinions on carbon capture click here and here!
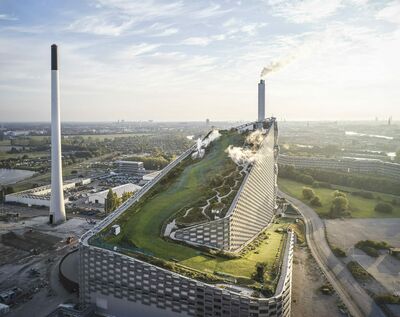
Relevance for WtE
CCSU is especially relevant for WtE since it can allow the sector to become carbon negative. “WtE combined with CCS becomes a carbon dioxide removal technology like direct air capture,” Tom Croymans explains. “Climate experts point out that carbon dioxide removal is a requirement to limit global warming to below 1.5°C. WtE has a crucial role to play here. WtE facilities typically treat on the order of several hundred thousand tonnes of waste each. By deploying CCS, we can start extracting CO2 from the atmosphere at a large scale in a short time.”
In addition to the CC technologies discussed so far, direct air carbon capture (DACC) is also being researched. However, at present, the majority of DACC projects are in the early stages of development, as Bruno Gerrits points out: “And atmospheric CO2 is dilute and can be difficult to capture, compared to industrial CO2
capture.” In addition, these technologies still require a lot of renewable energy. “Secondly, the CO2 concentration in the air is at the ppm level, while the CO2 concentration in WtE flue gases is approximately 10%,” says Tom Croymans. “And thirdly, the cost per captured tonne of CO2 is around $500 for DAC, while for WtE this is around a factor 10 lower.”
The captured CO2 can be used as an input for a range of products and services, whether it is used directly, e.g. as a solvent or heat transfer fluid, or after conversion, for example in the chemical industry
Enough storage space?
If not used on site, the captured CO2 has to be compressed and transported by pipeline, ship, rail or truck to either get it to production sites or to inject it into deep geological formations. “Storing CO2 involves the injection of captured CO2
into a deep underground geological reservoir of porous rock overlaid by an impermeable layer of rocks, which seals the reservoir and prevents the upward migration or ‘leakage’ of CO2 to the atmosphere,” says the IEA. Deep saline formations and depleted oil and gas reservoirs have the largest capacity. According to the agency there is enough underground storage globally, although further assessment work is required in some regions. And of course the necessary infrastructure must be set up – most WtE plants, for example, are not close to storage places.
By deploying CCS, WtE can start extracting CO2 from the atmosphere at a large scale in a short time.Tom Croymans, ESWET
A bright future?
Climate targets combined with stronger investment incentives are giving CCUS a new momentum. Nevertheless, a solid regulatory framework is needed to ensure the successful implementation of CCUS, says Tom Croymans: “Clear rules on the certification of captured carbon are also necessary, especially the recognition of the biogenic share of WtE emissions and the potential for the sector to be carbon negative. Access to funding and public support is also pivotal to contribute to the uptake of new projects and accelerate the deployment of these crucial technologies for the decarbonisation of the European industrial sector.”