WtE and Carbon Capture : 10+1 things to know about CCUS and Waste-to-Energy
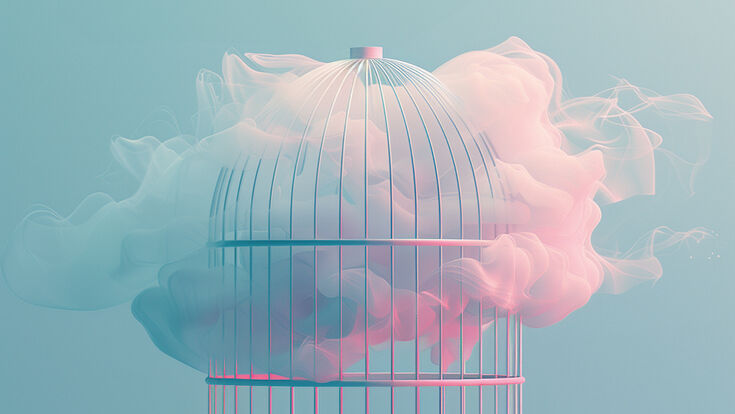
1. CCUS is not a technology per se but a variety of technologies
When talking about carbon capture (CC), it is essential to realise that implementing this system cannot stop at just installing a CC unit at the end of the flue gas cleaning line. This, as Fabio Poretti, Technical & Scientific Officer at the Confederation of European Waste-to-Energy Plants (CEWEP), points out, represents only the first two letters of the acronym.
The intermediate process, meaning transportation, is very often forgotten. "This aspect should not be taken for granted because CO2 needs to be compressed nearby the WtE site and typically also liquified, according to the logistic modality available in the territory," he explains. The transportation of the CO2 can then occur either via pipelines, trucks, trains or ships.
The ultimate destination of the captured and transported CO2 comes via permanent storage (Carbon Capture and Storage, CCS), or the CO2 is used as a valuable feedstock in a variety of applications (Carbon Capture and Utilisation - CCU). "A holistic approach to CCUS is hence essential, understanding the Technology Readiness Levels (TRL) for each step, as these vary along the value chain," says Poretti.
In terms of actual carbon capture technologies, for post-combustion CO2 capture, absorption using a solvent-based process is currently the most widely used and economically viable option. "Amine-based solvents have already been applied in large-scale commercial facilities in other sectors," says Fabio Poretti. Other post-combustion CO2 capture solutions available with an absorption process are HPC (Hot Potassium Carbonate) and enzyme-based capture.
Related article: "We believe CCUS will be a license to operate for WtE in the future."
Other CC techniques include adsorption processes, the use of membranes, oxy-combustion, Calcium Looping or more generally Chemical Looping Combustion (CLC). "The latter in particular generally have a lower TRL and they can be considered more as emerging technologies at the moment," says the expert.
Here’s a quick overview of how carbon capture works: Using conventional post combustion technology, the solvent traps the CO2 contained in the WtE flue gas within its molecular structure in the absorber column. The CO2-rich solvent then enters the desorber (or stripper) column where it is heated. This is the most energy-intensive part of the process. Here, the CO2 is boiled off, released at the top of the column and trapped separately. Finally, the lean solvent is returned to the absorber column to repeat the CO2 capture process over and over again.
Related article: “WtE must be part of a total waste management system”
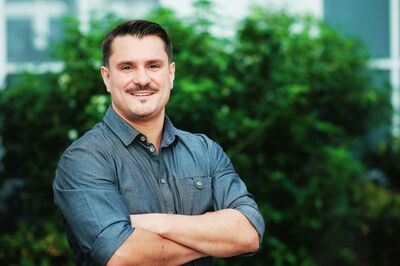
2. There are different solutions for storage and utilisation
Storage
CO2 can be stored underground in various types of deep geological formations (onshore or offshore). This technique involves converting CO2 into a high-pressure liquid and injecting it directly into sedimentary rock. The rocks may be located in depleted oil or gas fields, or in saline formations that act like a giant sponge. Various physical structures then prevent the CO2 from escaping to the surface.
On a global scale, storage capacity appears to exceed the need for net-zero emissions, but large-scale implementation will require significant investment and coordinated planning.
From a waste management perspective, CCS is a disposal operation because the CO2 is buried underground, essentially forever, and is not recovered. On the other hand, from a climate perspective, this also means it is not released back into the atmosphere. (see Figure 1)
Related article: "I envision a future where every WtE is equipped with a CCUS facility"
Utilisation
The market for CO2 utilisation is currently small and limited but has potential for growth. The main potential uses for CO2 that gained more attention recently are for the production of synthetic fuels, as they can be used in existing infrastructure and engines without major modifications, chemicals and plastics.
However, the production of new synthetic hydrocarbons also requires hydrogen, which is not readily available in nature. "There is no free lunch in thermodynamics," says Fabio Poretti. Hydrogen also requires energy to be produced, for example by breaking down H2O via water electrolysis.Considering the volumes of CO2 that could potentially be captured, a lot of hydrogen would be needed. This hydrogen should come from renewable sources in order to deliver net climate benefits, but renewables are also not available everywhere in unlimited quantities.
The mineralisation of CO2 is another interesting application that can be seen as an intermediate or fusion between CO2 storage and utilisation. The captured CO2 reacts with naturally occurring minerals such as iron (Fe), magnesium (Mg) and calcium (Ca). This is called 'mineral carbonation' and is a natural process that occurs over time as rocks weather. This process can be enhanced on an industrial scale. In this way, the captured carbon would remain chemically and permanently bound, and therefore stored, in the mineralised CCU product.
CCU valorises CO2, so from a circular economy perspective, it's more sound and an alternative way to recycle carbon. But from a climate viewpoint, in most CCU applications CO2 is released at a later stage (e.g. when synthetic fuels are combusted). In other words, some CCU applications would simply represent delayed CO2 emissions (somewhere else). Conversely, CCU applications may be valid even if they represent a delay in emissions, because they could result in overall climate savings from an LCA perspective, for example by avoiding the extraction of fossil fuels.
This the case for example in the Netherlands, where the use of greenhouses in the horticulture industry has been historically large and well developed. Although a plant (vegetable, flower, etc.) can only partially absorb the supplied CO2 via photosynthesis, the re-use of captured CO2 from WtE in greenhouses replaces the use of natural gas, saving an equivalent amount of fossil CO2 emissions. (see Figure 2)
3. There are two primary sources of carbon dioxide emissions in WtE processes
As a rule of thumb: The combustion of 1 tonne of residual waste in a conventional WtE plant generates approximately 1 tonne of total CO2 emissions at the stack.
However, the CO2 generated by WtE needs to be divided into two categories, depending on its origin:
- Fossil CO2, mainly from the incineration of fossil-based waste, such as residual plastics, textiles of fossil origin, etc.
- Biogenic CO2, which comes from the biodegradable fraction of various waste streams, such as paper and cardboard waste, wood, leather, kitchen waste and green waste that is not suitable for separate collection and recycling.
According to the CEWEP Climate Roadmap, the average share of biogenic CO2 emissions monitored at European level from WtE plants is around 60%, while the remaining 40% is fossil. Ultimately, the share of fossil and biogenic CO2 depends case by case on the composition of the residual waste delivered to the WtE plant.
The installation of a carbon capture unit at the end of a waste incineration line would allow the capture of fossil as well as biogenic CO2 emissions without distinction, proportional to the size (capacity) of the CC unit.
CCS can provide major savings in the climate change impacts of WtE.Fabio Poretti, CEWEP
4. WtE can benefit from implementing CCUS...
Integrating carbon capture and storage (CCS) solutions into existing municipal WtE plants offers significant improvements in climate impact without compromising other environmental impacts, according to a study by the Technical University of Denmark (DTU). "The full LCA modelling clearly showed that CCS can provide major savings in the climate change impacts of WtE despite the energy penalty that the technology has on the energy recovery of the WtE plant," says Poretti.
To put it another way, implementing CCU reduces the climate change impact of WtE and at the same time provides carbon for a range of uses. "However, life cycle assessment (LCA) shows that technology choices and the benefits of CCU applied to WtE depend on the energy system in which the WtE plant operates throughout its lifetime, and on the markets for the CCU products," says Fabio Poretti.
5. ...but there are various challenges as well
Technical challenges in relation to the CC unit itself
- The size of the carbon capture unit requires a certain amount of space.
- Energy penalty: energy consumption per tonne CO2 captured + heat/power requirements of the CO2 capture and CO2 conditioning unit. Heat integration is key. The energy loss makes less energy produced by the WtE plant available to the electricity grid or for local district heating and/or steam supply. Fabio Poretti: "It’s important to quantify and compare the net climate savings of the overall energy system where the WtE plant is located, with and without CCUS." Coupling carbon capture with flue gas condensation can represent a winning solution or in some cases an inevitable configuration to compensate the huge energy loss.
- Solvent management and impurities control
Challenges beyond the CC unit
- Projects take time
- Appropriate business models or funding schemes are essential
- Cost: Capex and Opex are still very high, especially for full-scale carbon capture plants. The energy crisis of recent years and the increase in all commodity prices have further delayed the realisation of some projects.
- Lack of policy and regulatory framework
- Lack of overall CO2 infrastructure and markets
- Community engagement and public perception are key. CCUS technologies also suffer from NIMBY (Not in my Backyard) syndrome.
Fabio Poretti: "Without forgetting the technical limitations described before, the real challenge is not within the boundary of the WtE plant and its CC unit: it’s a matter of making carbon capture competitive, logistically viable and economically affordable over the whole value chain."
The real challenge is not within the boundary of the WtE plant and its CC unit: it’s a matter of making carbon capture competitive, logistically viable and economically affordable over the whole value chain.Fabio Poretti, CEWEP
6. The location of WtE plants often makes the implementation of a CC unit impossible
The footprint is one of the main constraints and technical challenges. The space requirement should take into account not only the CO2 capture plant, but also the CO2 processing unit (including cooling, liquefaction, storage tanks), while maintaining safe distances.
It's important to remember that WtE plants are often located in cities or densely populated urban areas, so additional space near the plant may be very limited. "In this sense, carbon capture is not a silver bullet and won't be applicable to all WtE plants," says Poretti.
So while there have been (and will continue to be as technology advances) more compact solutions, footprint will remain a critical issue, especially for larger ambitions.
7. Interest in CCUS is increasing across different industries and countries
According to the Global CCS Institute, there are already 669 commercial CCS facilities worldwide. Most of them are in the US (275). WtE comes in tenth on the list of industry sectors most interested in implementing CCS technology. (see Table 1 and 2)
In Europe, the Netherlands and Norway have been at the forefront of research and industrial-scale experimentation for many years now. Interest has recently been accelerating in the UK.
At EU level, the role of WtE was also recognised when the European Commission published its long-awaited Communication on Industrial Carbon Management, also known as the EU CCUS Strategy, on 6 February. The strategy is a roadmap that identifies a holistic set of actions to be taken at EU and national level to create a single market for CO2 in Europe over the coming decades. The Strategy does not include a legislative proposal. However, it lays the groundwork for the deployment of CCS, CCU and carbon removal technologies and places CCUS high on the EU political agenda.
Furthermore, the EU institutions are about to finalise the first EU-wide voluntary framework for the certification of carbon removal, which will be indispensable to reaching net zero in 2050.
The EU Commission must also assess the possibility of integrating carbon removals into the EU Emissions Trading System (ETS) by 2026 with the aim of expanding their markets.
Table 1: TOP 10 COUNTRIES with the Highest Number of Commercial CCS Facilities in the World | ||
---|---|---|
United States | 275 | |
United Kingdom | 74 | |
Canada | 62 | |
China | 24 | |
Norway | 23 | |
Denmark | 19 | |
Netherlands | 17 | |
Australia | 17 | |
Indonesia | 17 | |
Sweden | 12 |
Table 2: THE INDUSTRY SECTORS most interested in implementing CCS (carbon capture and storage) Technology | |
---|---|
CO2 Transport/Storage | 224 |
Hydrogen/Ammonia/Fertiliser | 87 |
Biofuels/Ethanol | 80 |
Power Generation and Heat | 79 |
Natural Gas Processing | 50 |
Cement/Lime | 28 |
Chemical | 25 |
Various/Yet to be confirmed | 26 |
Direct Air Capture | 21 |
Waste/Biomass to Power and Heat | 18 |
Oil Refining | 17 |
Iron and Steel | 6 |
Biomass to Power and Heat | 4 |
Pulp and Paper Production | 2 |
Renewable Hydrogen | 1 |
Aluminium | 1 |
Grand Total | 669 |
8. There are three WtE plants with existing carbon capture units on a commercial scale
Of the approximately 2,600 WtE plants worldwide, 500 of which are in Europe, there are currently three WtE plants equipped with an existing CC unit on a commercial scale: AVR WtE in Duiven (the Netherlands), Twence WtE in Hengelo (the Netherlands) and the Saga City WtE plant in Japan.
Nevertheless, there are around 60 active CCUS projects and initiatives in WtE plants across Europe, according to CEWEP. (Poretti et al.) One example at an advanced stage is the CCS project at the Klemetsrud WtE plant in Oslo.
Many more feasibility studies, pilot projects and initiatives are regularly announced and the momentum is growing.
AVR WtE in Duiven, the Netherlands: In Duiven, 120 km east of Rotterdam, CO2 is captured at the AVR WtE plant and delivered by truck to the horticulture industry. Re-used CO2 substitutes the use of natural gas in greenhouses for the cultivation of flowers, vegetables and other plants. The CO2 capture system was commissioned in August 2019 and has the capacity to capture 100,000 tonnes of CO2 per year.
Twence WtE in Hengelo, the Netherlands: In Hengelo, at the Twence WtE plant, a large-scale CC facility has recently been completed, with the capacity to capture 100,000 tonnes of CO2 annually on one line of the WtE plant. Twence previously also performed tests with a pilot project on-site capturing and transforming CO2 into sodium bicarbonate (baking powder). This was among the first installations in the world to test 'mineralisation' of CO2 for circular re-use in residual waste treatment.
Saga City WtE plant, Japan: Commissioned in 2016, the CCU facility captures CO2 from the WtE plant’s flue gas which is then utilised to cultivate crops and create algae cultures only a few hundred metres away, producing exotic body lotions and anti-ageing creams which are exported to retailers around the world.
The CCU unit treats approximately 5% of total WtE flue gas with a CO2 removal target of between 80% and 90%, corresponding to about 10 tonnes of CO2 captured per day. The CO2 capture technology supplied is chemical absorption based on a proprietary amine solvent.
9. The economic feasibility for CCUS and WtE depends on various factors
Economic feasibility is complex to assess and depends on many interrelated factors, for example
- the size chosen for the carbon capture unit (i.e. how much CO2 is to be captured),
- the context in which the WtE plant is located and
- the financial framework conditions
"Implementing CCUS at a WtE plant is not impossible, but concluding a business plan remains very challenging at the moment," says Fabio Poretti.
Without a proper funding mechanism at local, national or EU level or a solid business case, investment in CCUS may not be economically sustainable.
In addition, a number of uncertainties related to the existing lack of CO2 logistics infrastructure as well as political and regulatory uncertainties mean that investment security is not fully guaranteed. A business plan for a CCS project may be completely different from one for a CCU project. In the case of CCU, for example, another variable may come into play, namely access to green and cheap hydrogen in the case of the production of synthetic fuels or chemicals from CO2.
Another key parameter that strongly influences the economic viability of a project is the distance and access to a CO2 storage hub or industrial cluster for CO2 utilisation and conversion.
Countries located near the coast and with a nearby shipping terminal would have easier access to CO2 transport and storage possibilities. This could also generate unequal competition, for example at EU level among different Member States.
10. There will be more and different technologies in the future
"Carbon capture is not a new technology. We have been talking about it for decades. The difference from the past, however, is that a strong political driver could now bring it into a new era," says Poretti. "I believe we will keep expanding the offer of carbon capture equipment. Possibilities that are currently available only at lab scale at low TRL could become feasible in the future if the right conditions are in place, just as other solutions now commercially available were in their infancy some years ago."
+ 1 Waste-to-Energy is already a decarbonisation technology
In many regions, including Europe, the priority in the coming years will probably be investing in integrated waste management and reducing landfill, rather than CCUS. While CO2 emissions are often the focus, methane (CH4) is a greenhouse gas far more potent than CO2 ‒ 28 times more over 100 years and 86 times more over 20 years. Poorly managed landfills are major methane emitters. Diverting waste from landfills to higher steps in the waste hierarchy such as recycling and WtE can significantly cut methane emissions and yield greater CO2 equivalent savings than carbon capture technologies. Fabio Poretti: "It’s vital to adopt a holistic approach where CCUS can be seen as an extra and complementary solution that can further reduce the carbon footprint of an integrated waste management system."